CICONIIFORMES Bonaparte, 1854
![]() |
Click for Ciconiidae species tree |
---|
Although Hackett et al. (2008) found that the storks were basal in the remaining Ardeae (after the penguins and seabirds), Gibb et al. (2013) placed them next to the herons. However, Jarvis et al. (2014) came up with a different arrangement of the taxa, casting doubt on the Gibb et al. treatment. The latest analyses are from Prum et al. (2015) and Kuramoto et al. (2015), who both found the storks to be basal in this group.
Slikas (1997) did not come to a definitive conclusion on how to arranged the genera of the Ciconiidae. I've adopted her maximum likelihood tree. However, it may not be correct, and there were indications that Ciconia itself may not be monophyletic.
Ciconiidae: Storks Sundevall, 1836
6 genera, 19 species HBW-1
- Marabou / Marabou Stork, Leptoptilos crumenifer
- Lesser Adjutant, Leptoptilos javanicus
- Greater Adjutant, Leptoptilos dubius
- Abdim's Stork, Ciconia abdimii
- Woolly-necked Stork, Ciconia episcopus
- Storm's Stork, Ciconia stormi
- Black Stork, Ciconia nigra
- Maguari Stork, Ciconia maguari
- White Stork, Ciconia ciconia
- Oriental Stork, Ciconia boyciana
- Jabiru, Jabiru mycteria
- Black-necked Stork, Ephippiorhynchus asiaticus
- Saddle-billed Stork, Ephippiorhynchus senegalensis
- Asian Openbill, Anastomus oscitans
- African Openbill, Anastomus lamelligerus
- Wood Stork, Mycteria americana
- Yellow-billed Stork, Mycteria ibis
- Milky Stork, Mycteria cinerea
- Painted Stork, Mycteria leucocephala
SULIFORMES Sharpe 1891
AOU officially adopted the term Suliformes in the 51st supplement. Sharpe had previously used Sulae as a suborder. For that matter, he also had Fregatae and Phalacrocoraces as suborders. The Suliformes had previously been considered part of the Pelecaniformes, a tradition that dates back to their naming by Sharpe.
The Suliformes were traditionally considered part of the Pelecaniformes (as were the tropicbirds). After all, how likely was it that such unusual features as a totipalmate foot and gular pouch would arise independently? They also share the location of the salt-excreting gland and all lack an incubation patch. These similarities lead Linneaus to put all but the tropicbirds (which lack the gular pouch) in the same genus.
Fregatidae: Frigatebirds Degland & Gerbe, 1867 (1840)
1 genus, 5 species HBW-1
The frigatebird taxonomy follows Kennedy and Spencer (2004).
- Lesser Frigatebird, Fregata ariel
- Ascension Frigatebird, Fregata aquila
- Magnificent Frigatebird, Fregata magnificens
- Great Frigatebird, Fregata minor
- Christmas Frigatebird, Fregata andrewsi
Sulidae: Gannets, Boobies Wood, 1836
3 genera, 10 species HBW-1
![]() |
Click for Sulidae tree |
---|
Sulid taxonomy follows Patterson et al. (2011), which is similar to Friesen et al. (2002), except that Papasula is considered basal. The extinct Tasman Booby, often considered a separate species, is here considered a subspecies of the Masked Booby following Christidis and Boles (2008). More recently, Steeves et al. (2010) provides strong evidence for this treatment. They further argue that Sula dactylatra tasmani is identical with the still extant subspecies S. d. fullagari, in which case both should be referred to as S. d. tasmani.
- Abbott's Booby, Papasula abbotti
- Northern Gannet, Morus bassanus
- Cape Gannet, Morus capensis
- Australasian Gannet, Morus serrator
- Red-footed Booby, Sula sula
- Brown Booby, Sula leucogaster
- Blue-footed Booby, Sula nebouxii
- Peruvian Booby, Sula variegata
- Masked Booby, Sula dactylatra
- Nazca Booby, Sula granti
Anhingidae: Anhingas Reichenbach, 1849 (1815)
2 genera, 4 species HBW-1
Prum et al. (2015) estimated the split between the cormorants and anhingas occurred about 28 mya. It could have been earlier, as some estimates suggest it was over 45 mya. It couldn't be much later, as the oldest known fossil darter lived roughly 25 mya (Worthy, 2012). Although some DNA-based estimates put the split as far back as 47 mya, I think they make other taxa way too old, and have too many lineages surviving the Chicxulub impact that killed all the non-avian dinosaurs.
Within Anhingidae, the basal split is between the New World Anhingas and Old World Darters. Fossil evidence (Becker, 1986) suggests that happened at least 18 mya, and Kennedy et al. (2019a) estimate it at 18.4–22.5 mya. That suggests that the anhinga and darters shouldn't be in the same genus. They shouldn't even be in the same subfamily.
The name Notoplotus (Matthews, 1920), type novaehollandiae is available. With it, we can divide Anhingidae into two subfamilies, Anhinginae and Notoplotinae.
There has been a tendency to promote Anhingidae subspecies to species. There are two subspecies of Anhinga anhinga. Limited sampling by Kennedy et al. (2019a) found they separated roughly half a million years ago. This sort of separation usually indicates subspecies, not species, so we have only one species of Anhinga.
The same question can be asked of the subspecies of the darters. Unfortunately, Kennedy et al. (2019a) didn't test them, and I'm unaware of any papers that did. Kennedy et al. did examine DNA of the three darter species, finding that the Australasian Darter separated from the other two roughly 15 mya, and that the other two share a common ancestor around 10 mya.
The arrangement follows Kennedy et al. (2019a), which also provides genetic support for the four species treatment used here.
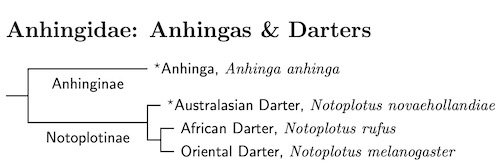
Anhingidae: Anhingas Reichenbach, 1849 (1815)
Anhinginae: Anhingas Reichenbach, 1849 (1815)
- Anhinga, Anhinga anhinga
Notoplotinae: Darters Informal
- Australasian Darter, Notoplotus novaehollandiae
- African Darter, Notoplotus rufus
- Oriental Darter, Notoplotus melanogaster
Phalacrocoracidae: Cormorants Reichenbach, 1849-50 (1836)
11 genera, 39 species HBW-1
The TiF treatment of the cormorants has changed over the years. Originally, I had used the DNA analyses of Kennedy et al. (2000, 2001, 2009) and the osteological analysis of Siegel-Causey (1988). The next version was primarily that of Kennedy and Spencer (2014). The latest version is based on Rawlence et al. (2022) and Kennedy et al. (2023). Don't let the two different names fool you. These two papers have 5 authors in common, including both of the named authors as well as Spencer.
![]() |
Click for Phalacrocoracidae species tree |
---|
Between them, the two recent papers cover all but two of the species given in recent checklists, and some of the subspecies. One troublesome species is the Japanese Cormorant, Phalacrocorax capillatus. Sangster and Luksenburg (2023) showed that the samples in Hondo et al. (2022) actually come from a Great Cormorant. I suspect the same is true of those used by Rawlence et al. (2022) and Kennedy et al. (2023).
The other missing cormorant is the recently extinct Spectacled or Pallas's Cormorant. Unfortunately, there are no tissue samples available from it. Unless DNA can be extracted from its bones, we will not be able to securely place it in the phylogeny.
It's thought that the nearly flightless Spectacled Cormorant was closely related to Compsohalieus and the two Urile species. That is where I have placed it, but in its own genus Pallasicarbo (Coues 1899), type perspicillatus. You can click on the tree diagram for the resulting phylogeny.
Two Inconsistent Time Scales
Both Rawlence et al. (2022) and Kennedy et al. (2023) give us dated phylogenies, which are helpful for determining how to divide the species into genera and subfamiles. Oddly, they did not attempt to reconcile the different dates used in the two papers, with Kennedy et al. putting the Leucocarbo-Nannopterum split at 8.6 mya and Rawlence et al. at 13.3 mya, 55% higher.
At the high end of the scale, Kennedy et al. are in close agreement with Prum et al. (2015), even though they compute the calibration differently. It wouldn't surprise me to find that the point estimates from Rawlence et al. (2022) are too high, and that the true numbers are nearer the low end of the HPD intervals. However, the estimates do seen rather reasonable for much of Leucocarbo.
General Organization of the Cormorants
Prum et al. (2015) estimated the Anhinga-Cormorant split occured about 28 mya, which is basically what Kennedy et al. (2023) found. Within the cormorants, the next split is between the smaller micro-cormorants and the rest, which occurred about 16.4 mya (±2.6). This is a good place to divide the cormorants into subfamilies—Microcarbinae and Phalacrocoracinae.
The initial division within the micro-cormorants happened about 12.2 mya, with the new genus Afrocarbo on one side (Kennedy et al., 2023), type coronatus, and both the monotypic Melanocarbo (Bernstein, 1883) and Microcarbo (Bonaparte, 1856), type pygmeus on the other. The split between Melanocarbo and Microcarbo dates to about 9.25 mya.
The other 8 genera will be used to organize the remaining cormorants. The Red-legged Cormorant, Poikilocarbo gaimardi, is basal in the Phalacrocoracinae, with the division between it and the others likely happening before 12.7 mya. The remaining cormorants now fall into two clades, with one paper estimating the split between them occurred 13.3 mya (!), and the other 8.6 mya. A number like 11 mya would be a reasonable guess, especially in view of the amount of subsequent speciation.
The first clade in the Phalacrocoracinae contains Brandt's Cormorant, now in the monotypic genus Compsohalieus (Baird, Brewer, & Ridgway 1884) and genus Urile (Bonaparte, 1855), type urile. These are grouped together with the uncertainly placed Spectacled Cormorant. The split between Compsohalieus and Urile seems to have occurred about 8 mya. We don't have a date for the extinct Spectacled Cormorant, which gets its own monotypic genus, Pallasicarbo (Coues, 1899). The remainder of the clade is in the much reduced genus Phalacrocorax (Brisson, 1760), type carbo, the Great Cormorant.
The Great Cormorant Complex
The Great Cormorant Complex includes all of the subspecies of Phalacrocorax carbo together with the White-breasted Cormorant, Phalacrocorax lucidus, which is often included in P.carbo.
I had previously thought the Japanese Cormorant, Phalacrocorax capillatus, should be included with them. Sangster and Luksenburg (2023) have shown that that was due to an erroneously identified DNA sample that was thought to be P.capillatus was used by Hondo et al. (2022). Based on Sangster and Luksenburg's analysis using the COI gene, I suspect the sample used in Kennedy and Spencer (2014), and subsequently in Kennedy et al. (2019b) and (2023) suffers from the same problem.
Its presence of P.capillatus in the middle of the Great Cormorant complex was the deciding factor when I split the complex. Due to Sangster and Luksenburg, I am undoing the split and returning to the IOC treatment where only the White-breasted Cormorant, P. lucidus is treated as separate from the Great Cormorant.
That leaves the following subspecies in the Great Cormorant, some of which may merit species rank:
- P.c. carbo which is a coastal bird in Europe and North America. Many of the birds in Britanny and Norway are genetically distinct, and have been referred to by the term `norvegicus`.
- P.c. sinensis ranges over much of Eurasia. In Europe, they tend to form colonies in trees near fresh water, while P.c. carbo nests on the coast. Recently, some of these colonies have contained both sinensis and carbo, especially in SE England. See Marion (1995), Marion and Le Gentil (2006), Goostrey et al. (1995), and Winney et al. (2001).
- The more greenish subspecies P.c. hanedae, which occurs in the Sea of Japan, has been thought to be very closely related to sinensis.
- P.c. novaehollandiae is present in Australia, New Zealand, and some other areas of Australasia.
- P.c. maroccanus occupies the NW African coast in Morocco and Mauritania. This form was not included in Kennedy et al.'s (2023) analysis.
Goostrey et al. (1995) used microsatellites, and were able to detect a signal of hybridization between sinensis and carbo when present in the same colonies in SE England. They also found birds that seemed intermediate. Marion and Le Gentil (2006) suggested that genetic changes were driven partly by the large increase in sinensis numbers. Whether the amount of hybridization is high enough to indicate both forms are the same species is unclear. At present, I just don't see enough evidence that there is enough hybridization to consider them a single species. Marion and Le Gentil did a back of the envelope calculation suggesting that the two lineages have been separated for almost 300,000 years. That's substantial, but not big enough to be sure they are two biological species, although they would species under many of the other species concepts.
It's a mistake to think that a bit of hybridization means that they are not species. Hybridization can continue for a long time. One cormorant example involves the Double-crested and Neotropic Cormorants. They have been separated for perhaps 4 million years (5 million according to Rawlence et al., 2022), they still hybridize.
To elaborate on the latter point: Several years ago we had an example of that at Wakodahatchee Wetlands in Delray Beach, FL. Several Neotropic Cormorants joined the Double-crested colony there, and one or two paired off with Double-crested Cormorants, successfully producing chicks that were intermediate in appearance. At least one of those subsequently mated with a Double-crested Cormorant.
Blue-eyed Shags: Cormorants of the Southern Ocean
The second clade contains three genera: Gulosus, Nannopterum, and Leucocarbo. Gulosus has a single species, the European Shag, Gulosus aristotelis. Needless to say, it is the type of the Gulosus (Montagu, 1813). The second genus is Nannopterum (Sharpe, 1899), type harrisi. It contains three species. The third genus, Leucocarbo, is the largest, containing over a dozen species.
Leucocarbo is also an impressive traveller. It has apparently used the strong westerlies of the Southern Ocean (Roaring Forties, Furious Fifties, Screaming Sixties) to travel east from South America all the way to New Zealand. Although that's the long way to travel there, it seems to be the path they took. All these cormorants, as well as their relatives that remained in South America comprise the genus Leucocarbo (Bonaparte, 1857), type bougainvilliorum.
One of the nice things about Rawlence et al. (2022) is that it's able to explain the distribution of blue-eyed shags, something that had previously puzzled me. Part of the puzzle is that blue-eyed shags have strong site fidelity. They don't stray very far, often staying within 15 mi. (25 km) of their nesting sites. Mainland blue-eyed shags (atriceps) don't even venture as far as Isla de los Estados, a mere 18 miles (28 km) off the Argentinian coast of eastern Tierra del Fuego. This behavior contrasts with migratory or partially migratory cormorants such as the Double-crested Cormorant or those in the Great Cormorant complex. Such birds may regularly travel 1000 mi. (1600 km) or more.
So the idea is that sometimes the shags venture too far, perhaps because they have lost their home, and the strong westerlies keep them from returning. In the days of sail, people referred to the Roaring Forties, Furious Fifties, and Screaming Sixties, indicating that the winds were strong and relentless, and got more so as you headed south. These winds could then carry the stray shags to the antarctic and subantarctic islands, or even to New Zealand -- and they did!
A second key to understanding the distribution of blue-eyed shags is that the subantarctic islands of the Indo-Atlantic region can sometimes be unsuitable as cormorant habitat, most likely during glacial maximia. Thanks to Keith Bennett (pers. comm.) for straightening me out on this. Mostly, it seems likely that the cormorants can survive these conditions, but in at least case, they apparently didn't.
In constrast, locations such as New Zealand and South American continued to provide sufficient cormorant habitat. Figure 1 in Rawlence et al. (2022), shows how the coromorants of the Indo-Atlantic islands, stretching from the Antarctic Peninsula, through the S. Shetlands, Marion Island, the Crozets, the Kerguelen Islands, Heard and MacDonald Islands, all the way to Macquarie Island, were more vulnerable.
Rawlence et al. (2022) provides a useful phylogeny of the blue-eyed shags. However, many of the nodes have low posterior probability. It appears that the use of nuclear genes has added noise, but little information. The supplementary material contains a phylogeny based on the mitochondrial genes. It is about the same, but unlike the other trees, posterior probabilities are high, mostlly 98% or better (Fig S1.1F).
The Shags Split
Roughly 2.5 mya (Rawlence et al. calibration) the shags split into a New Zealand clade and a South American/Indo-Atlantic Island clade. But here there is a puzzle, the New Zealand clade seems to have soon started to diversify. But the diversification of the South American clade all occurred within the last 650k years.
Surely the expansion to New Zealand left behind numerous shags on the Indo-Atlantic islands. But they seem to left no living progeny on anywhere from South America to Macquarie Island. Although the glacial maximum is usually not strong enough to do this (Keith D. Barnett, pers.comm), perhaps it was at least once. Or perhaps they succumbed to disease or inbreeding or some other cause.
South Island and Chatham Shags
Although the phylogeny of most of the New Zealand clade is clear enough, there is some complication involving the South and Chatham Island Shags.
The picture there prior to both Maori colonization was very different that it is now. Rawlence et al. (2015), showed that there were two lineages of shag on the South Island prior to the Marori arrival (ca. 1280). One, the Otago lineage, was widely distributed, and suffered a population crash of over 99%. The other, the Foveaux lineage, remained relatively stable. Rawlence et al. (2016) showed that the Foveaux shags are Leucocarbo stewarti and the Otago shags, L. chalconotus. Moreover, Chatham Island's L. onslowi was nested within the L. chalconotus clade (Fig. 4).
The ranges of the Otago and Foveaux shags abut. This is not enough to prove that they are separate species, because even the small gap between their colonies might be just large enough that two types of shags may not mix in their breeding colonies. However, its apparent persistence suggests these are separate species. The Chatham Island Shag (L. onslowi) is probably best interpreted as a subspecies of the Otago Shag (L. chalconotus) as ancient DNA has it nested with the Otago Shags.
Figure 4 in Rawlence (2016) shows the situation we would find if we were ornithologists visiting New Zealand around 1000 AD. There would be at least clades associated with L. chalconotus, one of them also containing L. onslowi, the Chatham Island Shag. They're much more closely related to each other than to L. stewarti. This means we would likely call them two subspecies. The L. chalconotus population crashed around 1380 AD by over 99%. Returning to Figure 4, we focus on the lines with black circles, two of onslowi and 3 of chalconotus. Because of the extinction, we see two separate taxa, taxa that a thousand years ago we would have recognized at subspecies, but we are now tempted to call species. I think we should resist that temptation.
S. America and Subantartic Islands
Here's a list of the subspecies, listed from west to east.
- atriceps: Southern Cone of S. America
- albiventer: Falkland Islands
- bransfieldensis: Antarctic Peninsula, S. Shetland Is.
- georgianus: S. Georgia I., S. Orkney Is., S. Sandwich Is.,
Shag Rocks (Scotia Sea) - melanogenis: Marion I., Prince Edward I., Crozet Is.
- verrucosus: Kerguelen Is.
- nivalis: Heard I.
- purpurascens: Macquarie I.
One tidbit from Orta (1992=HBW-1), which points both to the possibility of movement between islands (presumably from west to east) and suggests the island taxa may be interfertile: ``Occasional occurrence of birds with white wing bars exclusively in E. Kerguelen might suggest recent arrival of some individuals of P. melanogenis or P. nivalis, which might have hybridized with local birds.'' To give an idea of the distances involved, the nearest subantarctic islands to the west are the Crozet Island, about 870 miles (1400 km) away.
The Supplementary Material: Mitochondrial DNA
I have a problem with the the Indo-Atlantic branch branch of the Rawlence et al. (2022, Figure 1) phylogeny. A close look at the phylogeny for the Indo-Atlantic shags reveals that the Bayesian support numbers are quite low (they should be near 1.0 for an accurate phylogeny). There's no particular reason to believe it once you get past the Guanay Cormorant, L. bougainvilliorum. The only solid node after that is for the South America/Indo-Atlantic clade. Even the New Zealand nodes mostly have mediocre support.
Fortunately, the supplementary material gives us a way forward. It includes individual gene trees for each of the nuclear genes, and a combined mitochondrial tree. Although the nuclear trees for the blue-eyed shags are generally poorly supported, most of the mitochondrial tree (5 genes) is strongly supported. In fact, only 3 nodes have a posterior probability (i.e., after optimization) below 98%, and only the Crozet Island node causes us any difficulties.
![]() |
Indo-Atlantic Blue-eyed Shags, by Island |
---|
Excerpted and abstracted from Figure S1.1.F, Rawlence et al. (2022), supplementary material. |
You'll notice that there is a problem with melanogenis in the mitochondrial tree. The samples from Crozet Island are not sister to those from Marion Island (and I presume, the unsampled shags of nearby Prince Edward Island). Consulting the nuclear gene trees yields no clear pattern. Sometimes melanogenis is a clade, sometime part of a grade, and you see what happens for the mitochondrial DNA. For now, I'm considering the melanogenis from Marion and Prince Edward Islands as unnamed subspecies of bransfieldensis, not melanogenis. Those from the Crozet Islands become the Crozet Shag, L. melanogenis.
Rawlence et al. (2022) found L. chalconotus and L. onslowi separated about 300,000 years ago, and I'm using this as a rough standard for evaluating splits, provided there is no other convincing evidence. So what do we have?
When applied to the mitochondrial phylogeny of Rawlence et al., the following taxa count as species. First, the New Zealand group: ranfurlyi, carunculatus, chalconotus, stewarti, campbelli, and colensoi. Second, we have the Indo-Atlantic group: atriceps, albiventer, georgianus, melanogenis (Crozet), and purpurascens.
Some key points:
- I've lumped the Chatham Shag, Leucocarbo onslowi into the Otago Shag, Leucocarbo chalconotus.
- The Foveaux Shag, Leucocarbo stewarti has been separated from Leucocarbo chalconotus.
- The Crozet Shag, Leucocarbo melanogenis, is restricted to the Crozets.
- The Heard Island Shag, Leucocarbo nivalis, Kerguelen Shag, Leucocarbo verrucosus, and Macquarie Shag, Leucocarbo purpurascens, have been merged as the Subantarctic Cormorant, Leucocarbo purpurascens.
- The shags from the Prince Esward Islands are considered an unnamed subspecies of the Antarctic Shag, Leucocarbo bransfieldensis.
- The Antarctic Shag, Leucocarbo bransfieldensis, and S. Georgia Shag, Leucocarbo georgianus, are considered separate species from each other and the Imperial Cormorant, Leucocarbo atriceps.
Phalacrocoracidae: Cormorants
11 genera, 40 species
Microcarbinae: Microcormorants
- Long-tailed Cormorant / Reed Cormorant, Afrocarbo africanus
- Crowned Cormorant, Afrocarbo coronatus
- Little Pied Cormorant, Melanocarbo melanoleucos
- Little Cormorant, Microcarbo niger
- Pygmy Cormorant, Microcarbo pygmeus
Phalacrocoracinae: Cormorants, Shags
- Red-legged Cormorant, Poikilocarbo gaimardi
- †Spectacled Cormorant / Pallas's Cormorant, Pallasicarbo perspicillatus
- Brandt's Cormorant, Compsohalieus penicillatus
- Pelagic Cormorant, Urile pelagicus
- Red-faced Cormorant, Urile urile
- Bank Cormorant, Phalacrocorax neglectus
- Cape Cormorant, Phalacrocorax capensis
- Japanese Cormorant, Phalacrocorax capillatus
- Great Cormorant, Phalacrocorax carbo
- White-breasted Cormorant, Phalacrocorax lucidus
- Socotra Cormorant, Phalacrocorax nigrogularis
- Spotted Shag, Phalacrocorax punctatus
- Pitt Shag, Phalacrocorax featherstoni
- Indian Cormorant, Phalacrocorax fuscicollis
- Little Black Cormorant, Phalacrocorax sulcirostris
- Black-faced Cormorant, Phalacrocorax fuscescens
- Pied Cormorant / Australian Pied Cormorant, Phalacrocorax varius
- European Shag, Gulosus aristotelis
- Flightless Cormorant, Nannopterum harrisi
- Neotropic Cormorant, Nannopterum brasilianum
- Double-crested Cormorant, Nannopterum auritum
- Magellan Cormorant / Rock Shag, Leucocarbo magellanicus
- Guanay Cormorant, Leucocarbo bougainvilliorum
- Falkland Cormorant, Leucocarbo albiventer
- Imperial Cormorant / Imperial Shag, Leucocarbo atriceps
- South Georgia Shag, Leucocarbo georgianus
- Antarctic Shag, Leucocarbo bransfieldensis
- Crozet Shag, Leucocarbo melanogenis
- Subantarctic Shag, Leucocarbo purpurascens
- Bounty Shag, Leucocarbo ranfurlyi
- New Zealand Shag, Leucocarbo carunculatus
- Auckland Shag, Leucocarbo colensoi
- Campbell Shag, Leucocarbo campbelli
- Otago Shag, Leucocarbo chalconotus
- Foveaux Shag, Leucocarbo stewarti
PLATALEIFORMES Newton 1884
Although some analyses have indicated that the herons and ibises are sister clades, support has been weak. Gibb et al. (2013) studied the complete mitochondrial genome of 4 herons, 4 ibises, and related taxa. They found that the herons and ibises do not form a clade, and estimated that they have been separate lineages since the early Paleocene. Since there is uncertainty about their closest relatives, and each represents a truly ancient lineage, I treat them both in their own orders.
Kuramoto et al. (2015) have found evidence of early hybridization between ancient ibises and herons following the split of between the heron and pelican lineages. That would explain the conflicting results in earlier analyses.
Threskiornithidae: Ibises, Spoonbills Poche, 1904
13 genera, 35 species HBW-1
The traditional treatment of the ibises and spoonbills as sister subfamilies
is just wrong. The spoonbills are not the sister group of the ibises. Rather, they
are most closely related to Threskiornis and perhaps Pseudibis.
Krattinger's MA thesis (2010) shows this fact clearly. Chesser et al. (2010) is consistent
with this idea, and it already appeared in Sibley and Ahlquist (1990;
esp. Fig. 367). Oddly, Sibley and Ahlquist did not comment on it.
Perhaps they found it too unbelievable.
Interestingly, there had been other hints that the spoonbills should not be treated as a subfamily. Matheu and del Hoyo (1992=HBW-1) mention that the Eurasian Spoonbill has been known to hybridize with Black-headed Ibis,Threskiornis melanocephalus. Unfortunately, they did not make the connection with Sibley and Ahlquist's results.
Krattinger (2010) also estimated divergence times. His results suggest that the spoonbill clade originated about 15 million years ago (with large error bars). That time span is more than sufficient to evolve even such a distinctive bill. The Hawaiian Honeycreepers evolved theirs in half that time (Lerner et al, 2011).
Krattinger did find a deep division in Threskiornithidae, but it was between the exclusively New World genera (Eudociminae) and the rest (Threskiornithinae), not between the ibises and spoonbills. The treatment as subfamilies emphasizes this radical change in taxonomy of the ibises and spoonbills.
Krattinger (2010) examined DNA from just over half of Threskiornithidae. The exact position of some of the Old World genera was not conclusively resolved (Bostrychia, Lophotibis, Nipponia), but this tree is a reasonable interpretation of what Krattinger found. The resulting tree is also consistent with Chesser et al. (2010) and Sibley and Ahlquist (1990). Question marks indicate genera that were not sampled. The order within the spoonbills is based on Chesser et al. (2010), which included all of the spoonbills.
Current thinking is that the extinct Reunion Solitaire was actually an ibis! Moreover, it seems to have been closely related to the sacred-ibises (see Mourer-Chauviré et al., 1995). Accordingly, it appears at the head of Threskiornis.
You may think it odd that the family is called Threskiornithidae when Eudociminae is a much older name. The family was once referred to as Ibididae (based on Ibis Cuvier 1816), but the oldest use of the genus Ibis actually refers to the Mycteria storks. Ibididae had to be replaced, and everyone ultimately settled on basing it on Threskiornis, which replaced Cuvier's version of Ibis. Ultimately, the ICZN ruled on this (Opinion 1674) and the family is called Threskiornithidae.
Eudociminae Bonaparte, 1854
- White Ibis / American White Ibis, Eudocimus albus
- Scarlet Ibis, Eudocimus ruber
- Sharp-tailed Ibis, Cercibis oxycerca
- Green Ibis, Mesembrinibis cayennensis
- Bare-faced Ibis, Phimosus infuscatus
- Plumbeous Ibis, Theristicus caerulescens
- Buff-necked Ibis, Theristicus caudatus
- Black-faced Ibis, Theristicus melanopis
Threskiornithinae Poche, 1904
- Glossy Ibis, Plegadis falcinellus
- White-faced Ibis, Plegadis chihi
- Puna Ibis, Plegadis ridgwayi
- Crested Ibis, Nipponia nippon
- Madagascan Ibis, Lophotibis cristata
- Northern Bald-Ibis, Geronticus eremita
- Southern Bald-Ibis, Geronticus calvus
- Olive Ibis, Bostrychia olivacea
- Sao Tome Ibis, Bostrychia bocagei
- Spot-breasted Ibis, Bostrychia rara
- Hadada Ibis, Bostrychia hagedash
- Wattled Ibis, Bostrychia carunculata
- Roseate Spoonbill, Platalea ajaja
- Yellow-billed Spoonbill, Platalea flavipes
- African Spoonbill, Platalea alba
- Eurasian Spoonbill, Platalea leucorodia
- Black-faced Spoonbill, Platalea minor
- Royal Spoonbill, Platalea regia
- Red-naped Ibis, Pseudibis papillosa
- White-shouldered Ibis, Pseudibis davisoni
- Giant Ibis, Pseudibis gigantea
- Reunion Ibis, Threskiornis solitarius
- African Sacred-Ibis, Threskiornis aethiopicus
- Malagasy Sacred-Ibis, Threskiornis bernieri
- Black-headed Ibis, Threskiornis melanocephalus
- Australian White Ibis, Threskiornis molucca
- Straw-necked Ibis, Threskiornis spinicollis
PELECANIFORMES Sharpe 1891
The status of two monotypic families, the Shoebill and the Hamerkop, has been a perennial issue. The analyses of Ericson et al. (2006a) and Hackett et al. (2008) indicate that both are relatives of the pelicans. Indeed, they could all be lumped into the same family. We keep them separate not only because of their uniqueness, but also because the division between them seems to be ancient. Gibb et al. (2013) estimate that the pelican-shoebill split occurred in the early Eocene. According to Prum et al., the Shoebill is more closely related to the Pelicans and the Hamerkop is basal in the Pelicaniformes.
Scopidae: Hamerkop Bonaparte, 1849
1 genus, 1 species HBW-1
- Hamerkop, Scopus umbretta
Balaenicipitidae: Shoebill Bonaparte, 1853
1 genus, 1 species HBW-1
- Shoebill, Balaeniceps rex
Pelecanidae: Pelicans Rafinesque 1815
![]() |
Pelican species tree |
---|
The pelicans have been studied by Kennedy et al. (2013). The arrangement on the tree and order below reflects the relationships they found. Note how the New World Pelicans and Old World Pelicans form sister clades. They also found that the Pink-backed, Dalmatian, and Spot-billed Pelicans are quite closely related.
1 genus, 8 species HBW-1
- American White Pelican, Pelecanus erythrorhynchos
- Brown Pelican, Pelecanus occidentalis
- Peruvian Pelican, Pelecanus thagus
- Great White Pelican, Pelecanus onocrotalus
- Australian Pelican, Pelecanus conspicillatus
- Pink-backed Pelican, Pelecanus rufescens
- Dalmatian Pelican, Pelecanus crispus
- Spot-billed Pelican, Pelecanus philippensis
ARDEIFORMES Wagler, 1830
Ardeidae: Herons, Egrets, Bitterns Leach, 1820
21 genera, 82 species HBW-1
Like the other bird orders, Ardeiformes is old. Both Prum et al. (2015) and Kuhl et al. (2021) estimate the recent common ancestor for the Ardeiformes and their nearest relatives, the Pelecaniformes, lived about 52.5 mya (Eocene). This is a typical age for bird orders.
In the past, the Boat-billed Heron had been considered to be the a separate monotypic family, the Cochlearidae. DNA has made clear that it is really just another heron.
The list here is pieced together from the available DNA evidence. This includes the ultraconserved element analysis of Hruska et al.\ (2023), as well as Chang et al., 2003; ; Huang et al. (2016), Päckert et al. (2014), Sheldon et al., 2000; Zhou et al., 2014, 2016; and the trees posted to BirdForum by Raty in 2014 and 2023 I had previously constulted the traditional morphological evidence of McCracken and Sheldon (1998), but the DNA evidence is now complete enough that this is no longer necessary.
Heron / Egret genus tree |
---|
![]() |
Click for species tree |
The analysis by Hruska et al. (2023) has finally allowed me to construct a reasonably reliable genus tree for the herons, egrets, and bitterns. Species converage remains incomplete, but I was even able to put together a decent species tree. The Ardeidae bird list has been updated to match the species tree.
Ardeidae Subfamilies
The Tigriornithinae (tiger-herons) are the first subfamily in Ardeidae. They are followed by two monotypic subfamilies, the Cochlearinae (Boat-billed Heron) and Agamiinae (Agami Heron). After that are the Botaurinae (bitterns) including Zebrilus, Botaurus and Ixobrychus. Chang et al. (2003), Päckert et al. (2014), and Zhou et al. (2014, 2016) found the Black Bittern embedded in Ixobrychus. It has sometimes been put in the monotypic genus Dupetor, but that is clearly embedded in Ixobrychus.
All but three of the Botaurinae were considered by Päckert et al. (2014). The linear order here is based on a combination of their results and those of Hruska et al. (2023). When Päckert et al. was new, moving the Least Bittern, Ixobrychus exilis, into Botaurus was a novelty. Even though they look similar, it's not surprising that the Least Bittern is not sister to the Little Bittern group (I. minutus–novaezelandiae). The osteological evidence in McCracken and Sheldon (1998) had long ago indicated it is not as close to the Little Bittern group as one might think.
The Stripe-backed Bittern, Ixobrychus involucris, has been moved the other way, from Ixobrychus to the genus Botaurus. See See Päckert et al. (2014), Huang et al. (2016) and Hruska et al. (2023).
For a long time, it was unclear where all of the night-herons would fall out. With Hruska et al. (2023), that is no longer an issue. Two of the night-herons: White-backed Night-Heron, Calherodius leuconotus and White-eared Night-Heron, Oroanassa magnifica, are not part of the night-heron clade. However, the rest of the night-herons form a subfamily, Nycticoracinae.
The remaining genera, including the two special night-herons make up the last subfamily, Ardeinae. Ardeinae consists of three parts. The first centers on the genus Egretta, the second includes the green/striated and pond-herons. The third contains many of the larger herons and egrets, both light and dark.
Splits
The M.Sci. thesis by Mendales (2023) made clear that the New World and Old World Striated Herons should be separated. The New World version, which I'm calling the American Striated Heron, Butorides striata, is most closely related to the Green and Lava Herons, while the other forms are more distant.
However, Mendales also found structure in the Old World Striated Herons. How that works may need more complete taxon sampling to become fully evident. Nonetheless, I'm taking a stab at it anyway and going with 4 Old World Striated Herons based on plumage. That splits the Striated Heron, Butorides striata into five taxa:
- American Striated Heron, Butorides striata
- African Striated Heron, Butorides atricapilla
- Arabian Striated Heron, Butorides brevipes
- Asian Striated Heron, Butorides javanica
- Australasian Striated Heron, Butorides macrorhyncha.
The Cattle Egret, Bubulcus ibis, has been split into Western Cattle Egret, Bubulcus ibis, and Eastern Cattle Egret, Bubulcus coromandus based on differences in plumage and DNA (Raty barcode tree).
The Intermediate Egret, Mesophoyx intermedia, has been split into Intermediate Egret, Mesophoyx intermedia, Yellow-billed Egret, Mesophoyx brachyrhyncha (sub-Saharan Africa), and Plumed Egret, Mesophoyx plumifera (Australasia) based on differences in breeding plumage (HBW/BirdLife).
Kushlan and Hancock (2005) and Christidis and Boles (2008) suggested treating the Great Egret as two species: Casmerodius albus and Casmerodius modestus. Certainly, the genetic distance between some of the Great Egret subspecies is quite large, comparable to that between Great and Intermediate Egret (Sheldon, 1987), but the subspecies analyzed are egretta and modestus. This suggest no significant gene flow between egretta and modestus, that they are distinct biological species.
But how do the other subspecies (albus and melanorhynchos) fit in? Both Kushlan and Hancock, and Cristidis and Boles, suggest that egretta should be grouped with albus and melanorhynchos. However, Pratt (2011) argues that the split should be between egretta and the rest, mainly on the basis of breeding plumage. However, Raty's 2014 barcoding tree suggests that albus and egretta are more closely related to each other than to modestus (with low support). It also suggests a species level difference between albus and egretta. Further, all 4 subspecies (including melanorhynchos) have distinctive breeding plumages.
Accordingly, I've split the Great Egret, Casmerodius modestus, into 4 species, based on differences in breeding plumage and, except for melanorhynchos, DNA.
- Eastern Great Egret, Casmerodius modestus,
- Great White Egret, Casmerodius albus,
- African Great Egret, Casmerodius melanorhynchos, and
- American Egret, Casmerodius egretta.
The status of the Great White Heron, remains controversial (e.g., Stevenson and Anderson, 1994). Should we think of it as Ardea occidentalis or as Ardea herodias occidentalis? It is very near the borderline for species status. Genetically, it is nested within the larger Great Blue Heron clade. However, in their overlap zone in extreme south Florida, there seems to be very little interbreeding between the dimorphic Great White Herons (the dark morph is sometimes called Würdemann's Heron) and the monomorphic Great Blue Herons (McGuire, 2002; McGuire et al., 2019; Browning and Kushlan, 2022). Moreover, the Great Blue Herons of the Florida peninsula (wardii) are more closely related to those of the northern US (herodias) than to occidentalis. However wardii and herodias are closer to occidentalis than any of them are to fannini.
Starting with version 3.10, I've split the Great White Heron, Ardea occidentalis, from the Great Blue Heron, Ardea herodias. I had been on the fence about splitting them for some time. One thing that bothered me was how the two taxa could remain separate in spite of breeding in close proximity. I didn't appreciate that they used the method used by a number of seabirds. They don't breed at the same time of the year. This helps keep them separate, and has pushed me off the fence.
Ardeidae Species List
Tigriornithinae: Tiger-Herons Bock, 1956
- White-crested Tiger-Heron, Tigriornis leucolopha
- Rufescent Tiger-Heron, Tigrisoma lineatum
- Bare-throated Tiger-Heron, Tigrisoma mexicanum
- Fasciated Tiger-Heron, Tigrisoma fasciatum
Cochleariinae: Boat-billed Heron Chenu and des Murs, 1854 (1838)
- Boat-billed Heron, Cochlearius cochlearius
Agamiinae: Agami Heron Sangster, Kushlan, Gregory, and Dickinson, 2023
- Agami Heron, Agamia agami
Botaurinae: Bitterns Reichenbach, 1849-50
- Zigzag Heron, Zebrilus undulatus
- Stripe-backed Bittern, Botaurus involucris
- Least Bittern, Botaurus exilis
- Eurasian Bittern, Botaurus stellaris
- Australasian Bittern, Botaurus poiciloptilus
- American Bittern, Botaurus lentiginosus
- Pinnated Bittern, Botaurus pinnatus
- Black Bittern, Ixobrychus flavicollis
- Cinnamon Bittern, Ixobrychus cinnamomeus
- Von Schrenck's Bittern, Ixobrychus eurhythmus
- Dwarf Bittern, Ixobrychus sturmii
- Little Bittern, Ixobrychus minutus
- Yellow Bittern, Ixobrychus sinensis
- Black-backed Bittern, Ixobrychus dubius
- †New Zealand Bittern, Ixobrychus novaezelandiae
Nycticoracinae: Night-Herons Bonaparte, 1854
- Malayan Night-Heron, Gorsachius melanolophus
- Japanese Night-Heron, Gorsachius goisagi
- Yellow-crowned Night-Heron, Nyctanassa violacea
- †Bermuda Night-Heron, Nyctanassa carcinocatactes
- Nankeen Night-Heron, Nycticorax caledonicus
- Black-crowned Night-Heron, Nycticorax nycticorax
- †Ascension Night-Heron, Nycticorax olsoni
- †Reunion Night-Heron, Nycticorax duboisi
- †Mauritius Night-Heron, Nycticorax mauritianus
- †Rodrigues Night-Heron, Nycticorax megacephalus
Ardeinae: Egrets and Herons Leach, 1820
- White-backed Night-Heron, Calherodius leuconotus
- White-eared Night-Heron, Oroanassa magnifica
- Capped Heron, Pilherodius pileatus
- Whistling Heron, Syrigma sibilatrix
- Pied Heron, Egretta picata
- White-faced Heron, Egretta novaehollandiae
- Little Blue Heron, Egretta caerulea
- Tricolored Heron, Egretta tricolor
- Reddish Egret, Egretta rufescens
- Snowy Egret, Egretta thula
- Little Egret, Egretta garzetta
- Western Reef-Heron, Egretta gularis
- Dimorphic Egret, Egretta dimorpha
- Black Heron, Egretta ardesiaca
- Slaty Egret, Egretta vinaceigula
- Chinese Egret, Egretta eulophotes
- Pacific Reef-Heron, Egretta sacra
- American Striated Heron, Butorides striata
- Green Heron, Butorides virescens
- Lava Heron, Butorides sundevalli
- African Striated Heron, Butorides atricapilla
- Arabian Striated Heron, Butorides brevipes
- Asian Striated Heron, Butorides javanica
- Australasian Striated Heron, Butorides macrorhyncha
- Forest Bittern, Zonerodius heliosylus
- Rufous-bellied Heron, Ardeola rufiventris
- Squacco Heron, Ardeola ralloides
- Malagasy Pond-Heron, Ardeola idae
- Chinese Pond-Heron, Ardeola bacchus
- Indian Pond-Heron, Ardeola grayii
- Javan Pond-Heron, Ardeola speciosa
- White-necked Heron, Casmerodius pacificus
- Yellow-billed Egret, Casmerodius brachyrhynchus
- Intermediate Egret, Casmerodius intermedius
- Plumed Egret, Casmerodius plumiferus
- Eastern Great Egret, Casmerodius modestus
- Great White Egret, Casmerodius albus
- African Great Egret, Casmerodius melanorhynchos
- American Egret, Casmerodius egretta
- Western Cattle Egret, Bubulcus ibis
- Eastern Cattle Egret, Bubulcus coromandus
- Humblot's Heron, Ardea humbloti
- White-bellied Heron, Ardea insignis
- Great-billed Heron, Ardea sumatrana
- Purple Heron, Ardea purpurea
- Goliath Heron, Ardea goliath
- Black-headed Heron, Ardea melanocephala
- Gray Heron, Ardea cinerea
- Cocoi Heron, Ardea cocoi
- Great Blue Heron, Ardea herodias
- Great White Heron, Ardea occidentalis